Research
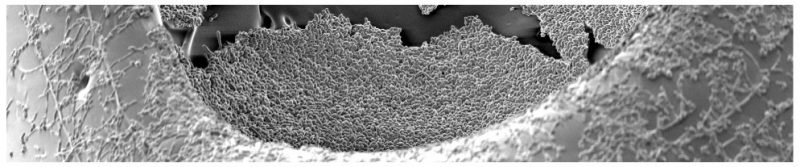
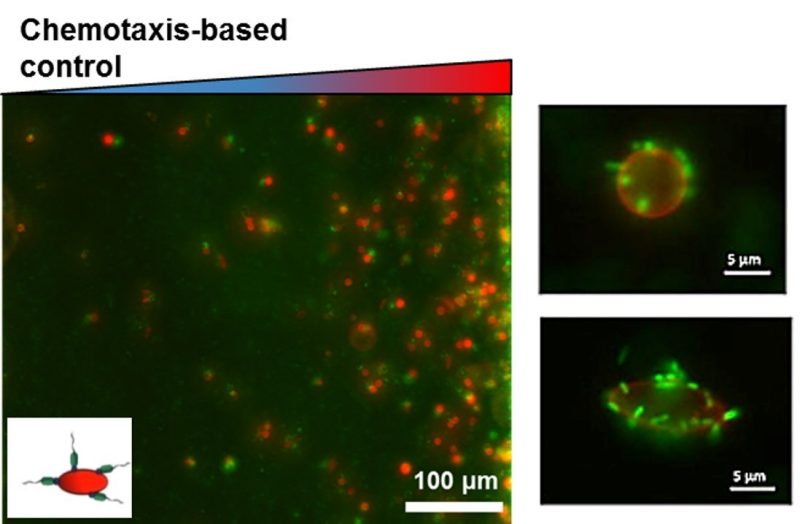
Bacteria-based Bio-Hybrid Micro-Robots (BacteriaBots)
We harness the sophisticated and robust machinery of bacteria for actuation, sensing, communication, and control in a class of micron scale (characteristic length: 2-100 µm) robotic systems called BacteriaBots. BacteriaBots are comprised of engineered micro/nano-particles of various sizes and shapes interfaced with live engineered bacteria. Utilizing methods from microrobotics, bioengineering, biology, and physical chemistry, many different aspects of such bio-hybrid systems such as the geometry of the robot body and bacterial phenotypic behaviors can be controlled for a wide variety of applications.
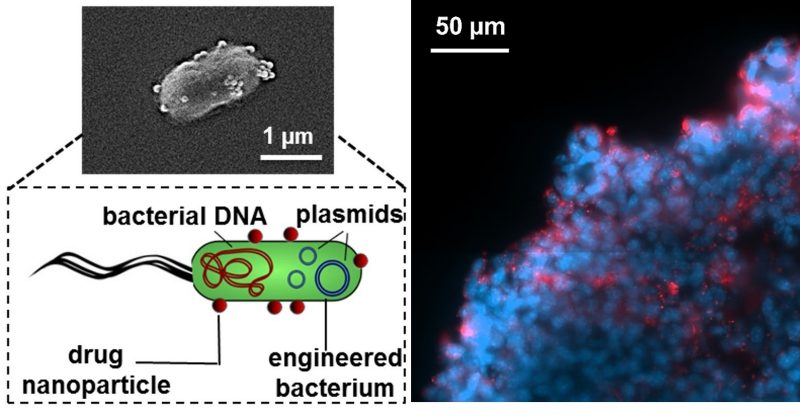
Nanoscale Bacteria-Enabled Autonomous Delivery Systems (NanoBEADS)
Systemic chemotherapy is the most common form of cancer therapy nearly all types and stages of cancer. Although limitations of chemotherapy have often been ascribed to drug resistance at the cellular level, there is substantial evidence suggesting that tumor microenvironment also mediates resistance of solid tumors to cancer therapy by limiting the drugs from penetrating in tumor tissue and reaching cells distal from blood vessels in sufficient concentrations. Bacteria-mediated drug delivery systems possess unique characteristics that can address these shortcomings. Several strains of attenuated bacteria, such as Escherichia coli, Salmonella Typhimurium have been identified to possess the natural ability to target and preferentially colonize tumor tissues. Our research focuses on the extravascular transport of tumor targeting bacteria. We aim to transform current practices and enable the development of active, controlled and resilient methods for targeted in-situ theranostics by harnessing the power of live attenuated tumor-targeting bacteria.
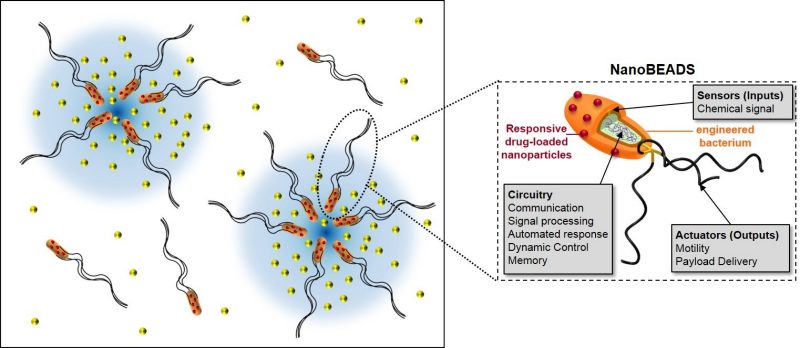
Distributed Control Strategies Inspired by Bacteria Population Dynamics
Mesoscale robotic systems are favored for a variety of applications including minimally invasive treatment of diseases, environmental monitoring, and reconnaissance. These miniature systems naturally have limited capabilities; thus, swarms of hundreds or more of such systems are needed to collectively accomplish a significant task. Simpler and cheaper agents will be critical to the feasibility of swarms with a large number of agents.
Biological cooperative communities can provide great insights into the development of novel and scalable mathematical strategies for robust control of robotic swarms. In particular, the study of bacteria population dynamics demonstrates that very simple control schemes can elicit relatively complex behaviors and that stochastic mobility and multiplicity can be effectively harnessed to scout large areas, cooperatively construct structures, and transport mass and energy over length scales thousands of times larger than each bacterium. We are currently investigating how the high gain sensory response of each individual agent combined with programmable communication and collaboration among agents affect collective swarm behavior in complex environments.
Bacteria-based Particle Sorting Platform
We developed a simple and cost-effective microfluidic sorting platform for the separation of similarly sized particles of dissimilar surface properties. Differences in surface chemistry between two groups of similarly sized nanoparticles in a mixture were exploited to selectively assemble one particle group onto motile E. coli, through either specific or non-specific adhesion, and separate them from the remaining particle group via chemotaxis (directed migration in response to a chemo-effector gradient) of the bacteria.
Emergent Behaviors of Microbial Communities
Bacteria play a central role in a wide array of disciplines ranging from microbiology to medicine to engineering. Many natural and engineered functions alike depend on emergent behaviors stemming from the collective, coordinated activity of individuals. One particularly interesting population-level behavior that is both ubiquitous in nature and a powerful tool for engineered microbial systems is quorum sensing (QS), whereby microbes secrete small signaling molecules called autoinducers (AIs) to communicate. When the concentration of AIs becomes high enough, a particular set of genes is expressed. We use agent-based models to elucidate, predict, and engineer this behavior in a spatiotemporal manner (top video). As interactions in diverse populations are omnipresent in nature, we characterize functionality not only in isogenic populations, but we also study and model interactions in diverse microbial communities (bottom image).
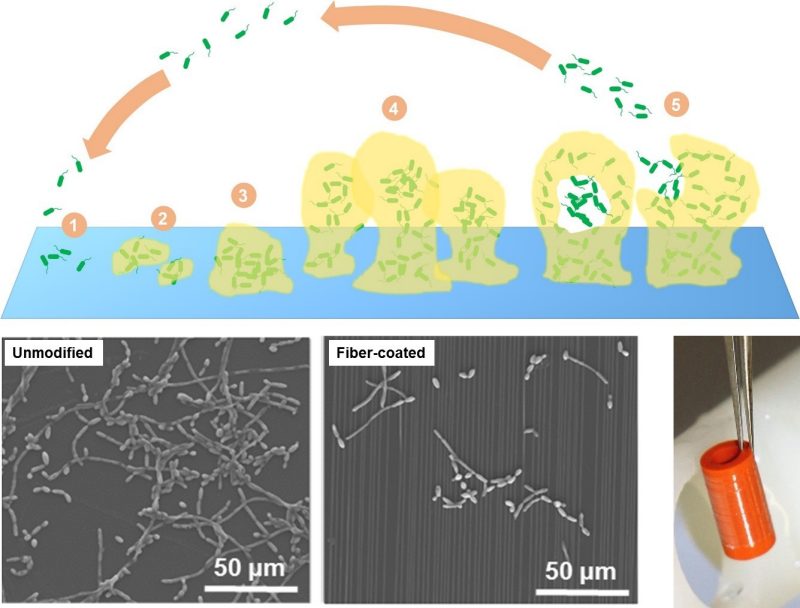
Microbial Surface Sensing and Adhesion
Microbial biofilms cause a significant portion of all human microbial infections. Nosocomial infections are the fourth leading cause of death and about 60–70% of nosocomial infections are associated with implanted medical devices. With advances in engineering biomaterials and regenerative tissue engineered therapies, we are likely to see an increase in the use of short and long-term biomedical implants. Current treatment paradigms for biofilm-associated infections typically consist of a combination of surgical replacement of the implant and long-term antibiotic therapy, which incur high health care costs and their application is likely to promote antibiotic resistance. Thus, there is a compelling need for new and efficient methods to mitigation of biofilm formation on implantable medical devices.
The objective of our research in this area is to understand the mechanisms of bacterial and fungal adhesion in order to develop methods of delaying and/or preventing infectious biofilm formation on medically relevant surfaces. Our current activities are focused on investigating the effect of submicron topographical cues on pathogen adhesion and biofilm formation.
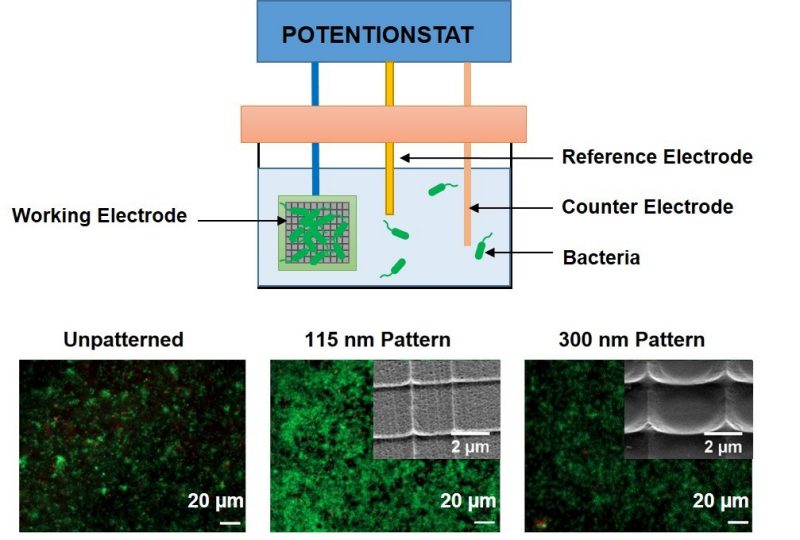
Electroactive Biofilms for Microbial Fuel Cells
Microbial fuel cells (MFCs) utilize electrochemically active microorganisms to oxidize organic fuels for electricity generation. MFC could serve as compact, portable and sustainable sources of energy for a broad range of applications including power production for field operations, remote monitoring, and miniature robots. However, applications are currently limited by low current and power density.
Our research focuses on understanding the effect of topography of the anode surfaces on the MFC performance. This effort will contribute to the critical understanding required to advance the science of microbial adhesion and electron transfer and develop enabling means to enhance the performance of MFCs.
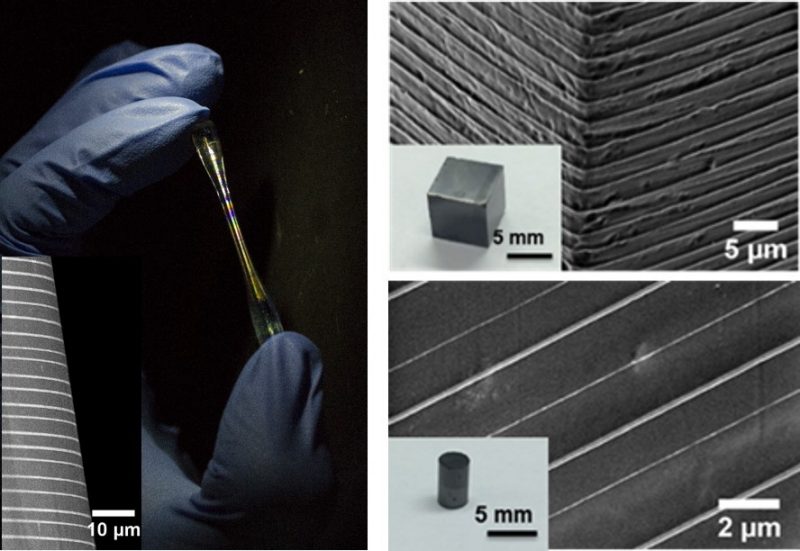
SWAN Lithography
We developed a versatile nanofabrication method called spun-wrapped aligned nanofiber (SWAN) lithography to pattern nanostructures of sub 50 nm to several microns on both planar and nonplanar 3D objects. This simple nanofabrication method offers high throughput and low capital cost and can be operated in standard laboratory settings. This technique has applications in the fields of photonics, plasmonics, electronics, and biotechnology.
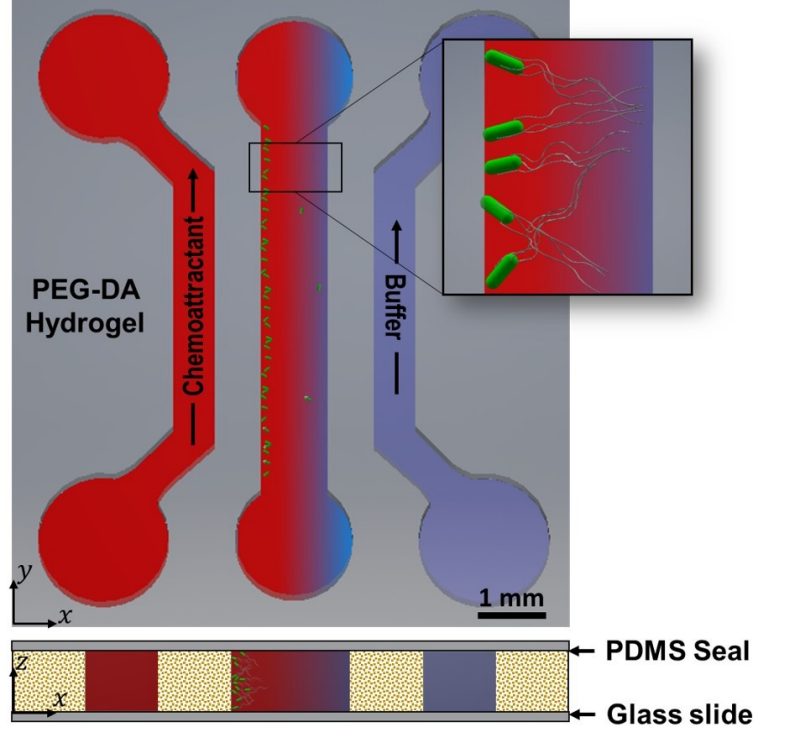
Biological Microfluidics
The response of prokaryotic and eukaryotic cells to chemical signals in chemotaxis, cell-cell communication (e.g., quorum sensing), and other processes are of significant importance in physiologically relevant processes such as wound healing and disease development such as infection or cancer metastasis. Our research in this area focuses on developing PDMS and hydrogel based microfluidic assay devices for the systematic study of cellular behavior in the presence of well-defined chemical and mechanical cues. We aim to elucidate the interplay of chemical, topographical and mechanical cues on cell behavior.
Supported by:
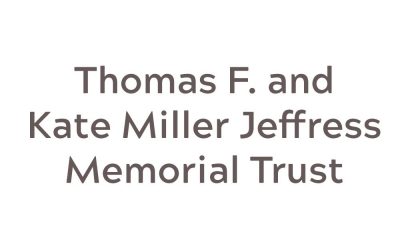
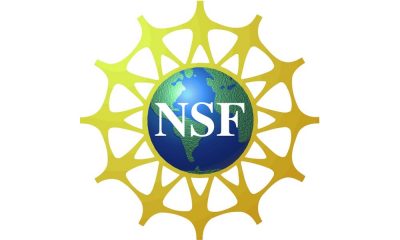
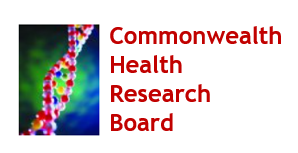
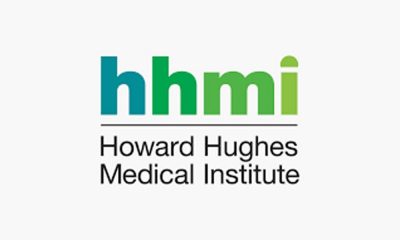
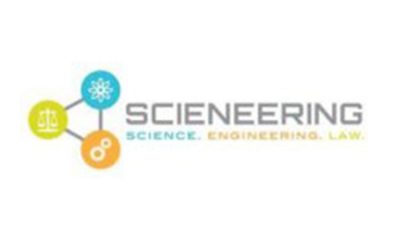
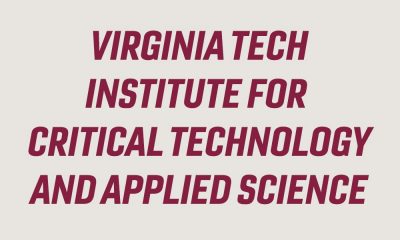